Transmitted Polarized Light Microscopy, Phase Contrast and DIC with the PureFocus850
Posted in PureFocus 850 Jan 24th 2024
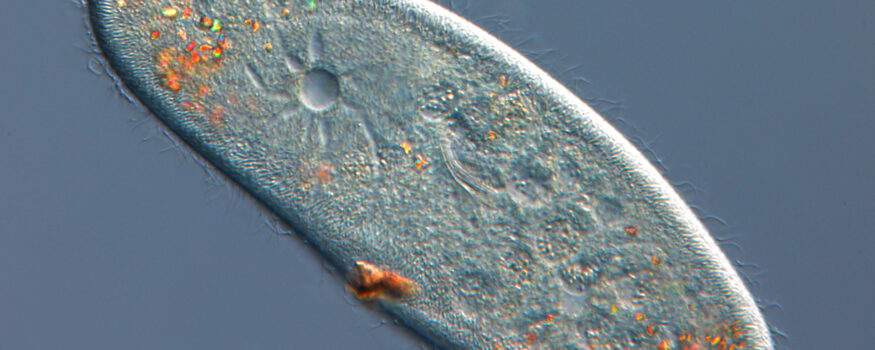
How does hardware autofocus adapt to specialized life science microscopy techniques?
In our previous article, we discussed the most routine life science microscopy techniques and how our hardware autofocus, the PureFocus850, can be used with these techniques and the benefits it can provide. This time, we explore other common but more specialized techniques that introduce more optical elements into the light path. While this might seem like a challenge for an optical device such as a hardware autofocus, we explain how the PureFocus850 has been designed with these techniques in mind, and how possible conflicts with these optical elements can be avoided.
Polarized Light Microscopy
Polarized light microscopy is a more specialized technique used for specific life science samples. It relies on a polarizer to linearly polarize white light transmitted through the sample to a specific orientation, and an analyzer (which is another linear polarizer) to select light that has had its polarization angle changed by the sample. Any light that has not been changed by the sample, or light from the environment surrounding the microscope, is blocked by the analyzer. Typically, the polarizer and analyzer are oriented orthogonally to achieve maximum extinction of any unchanged light. Light can be polarized in various ways – for example circular polarization – but for the purposes of this article we will give an explanation of linear polarization only.
What is linear polarization?
Light can be considered as a wave. Polarization refers to the direction of oscillation perpendicular to the direction of motion of the wave. White light is unpolarized – light waves are oscillating in all directions. A polarizer only allows light oscillating in a particular orientation to pass. So, in the context of a polarizing microscope, the sample is only illuminated by light with a particular polarization.
Samples used with polarization microscopy exhibit birefringence – linearly polarized light passing through the sample is ‘double-refracted’, producing two light rays that are polarized at 90° to one another. Components of these rays then interfere with one another when passing through the analyzer, enhancing the contrast of specific areas of the sample and causing them to acquire color depending on their properties. Picrosirius red is a stain used in pathology applications to detect fibrosis and causes collagen to appear red under polarized light. Polarization can also be useful for imaging unstained samples, for example when identifying gout crystals or when analysing petrological samples.
Is it possible to use hardware autofocus in polarized light microscopy?
Yes – as long at the autofocus system is set up to avoid interference from the microscope’s analyzer. The analyzer presents a problem because hardware autofocus systems use a laser source – which emits polarized light – to acquire focus. Many autofocus systems are mounted on the microscope camera port, where the autofocus laser source can be blocked by the microscope’s polarizer.
The PureFocus850, however, is mounted in the infinity space of a microscope, which makes it compatible with polarized light microscopy. For polarized light microscopy, it should be positioned between the objective and the analyzer to avoid interference. Most commercial microscopes will allow the analyzer to be mounted between the PureFocus850 and microscope camera or eyepieces.
Phase, relief and gradient contrast
Life science samples are typically transparent. Historically, stains were used to enhance the contrast between different sample features and the background. Adding stains is time consuming, degrades the sample and prevents imaging of live samples. Over time, alternative techniques to provide contrast evolved, such as phase contrast, relief (Hoffman modulation) contrast and gradient contrast.
Whilst these techniques use different components, they are underpinned by the same principle of converting phase differences of light passing through the sample into light intensity differences, enhancing the contrast observed by the microscopist. Phase contrast relies on the phase shift produced when light passes through the sample, and interference between the shifted and non-shifted light when the image is formed. Relief and gradient contrast rely on phase gradients present caused by differences in sample thickness in localised regions within the sample, causing light to be deflected into different areas of an optical component with variable transmission characteristics. Both techniques have the ability to highlight areas in the image where phase differences have occurred in contrast to the ‘background’, where no phase difference has been induced by the sample.
What is a phase difference?
Simply, a phase difference is produced when light passes from one medium (or refractive index) to another. Considering light as a wave, a change in the media the light is passing through changes the point in the light’s wave cycle. Cell features, such as membranes and other organelles, can be classified as different media. With light passing through these features changing phase, the optical elements used in each technique can now act on this light to produce contrast (differences in signal amplitude) that can be viewed by the microscopist.
None of the components specific to these techniques impact the functionality of the PureFocus850 as its laser light does not interact with them. Even with the enhanced contrast making camera-based autofocus more viable, the speed of a hardware-based autofocus is an asset for high throughput applications. As phase techniques are typically associated with live cell imaging and are now being used alongside artificial intelligence to characterize organelles and other intracellular features in the absence of fluorescence labelling or staining, the PureFocus850 can also support timelapse experiments or high content screening applications, where loss of focus can result in hours of wasted time.
Differential interference contrast
Differential interference contrast (DIC) uses aspects of phase microscopy techniques and polarized light microscopy to generate contrast in otherwise transparent or weakly contrasted samples. This technique features a complex optical path:
- Light emitted from the microscope illuminator is polarized to 45°.
- The polarized light enters a Nomarski-Wollaston prism and is separated into two rays polarized at 90° with respect to one another.
- The two rays pass through the sample but are sheared – they pass though slightly different points in the sample.
- This induces a difference in phase between the two rays where the sample differs in refractive index or thickness. The size of the phase difference depends on the magnitude of the refractive index or thickness difference.
- After passing through the sample, the rays are recombined into a single ray by a second Nomarski-Wollaston prism – the shear induced by the first prism is removed by the second. The recombined rays are still polarized in different orientations, so do not interfere.
- The recombined ray passes through an analyzer, which brings the polarization of both rays into the same plane, allowing them to interfere. In regions where a phase difference was induced by the sample, the rays interfere constructively or destructively. Where no phase difference has been induced, no interference occurs. This difference in interference across the image produces a 3D-like effect that highlights different features.
This interference produces an effect not unlike the phase microscopy techniques but avoids the bright halo artefacts typically associated with phase contrast. It also offers higher axial (Z-axis) resolution than the other techniques.
The PureFocus850 enables microscopists to take advantage of the high axial resolution of DIC by ensuring high fidelity to a specific focal plane while scanning over large areas or multi-well plates. As with setting up for polarized light microscopy, the PureFocus850 must be positioned between the objective and the analyzer, but the other components required – most notably the Nomarski-Wollaston prisms – do not interfere with its performance. Although PureFocus850 laser light passes through the prism twice, to and from the sample, the separation of the laser light and its recombination does not significantly impact the intensity of signal on the PureFocus850 sensor.
Interested in PureFocus850 for your application?
As illustrated in this article and previously, the PureFocus850 is compatible with the majority of life science microscopy techniques. Please get in touch with us to discuss your requirements or which microscope you would like to upgrade.
Is the PureFocus850 compatible with industrial microscopy techniques that use reflected light?
In this article we have focussed on transmitted light life science microscopy, but the PureFocus850 is absolutely compatible with reflected light microscopy techniques, including fluorescence as discussed previously. In a future article, we will explore how the PureFocus850 is a powerful tool for industrial microscopy when using reflected brightfield, darkfield, DIC and other techniques. You can read about how the PureFocus850 has greatly improved the throughput of wafer scanning here.